Our goal as pilots is to make every landing one you can walk away from. The goal of every plane designer is to make the structure as robust, repairable and survivable as possible.
With metal (primarily aluminum) airframes, the materials and design features are we’ll understood, while inspections and repairs are typically very straightforward. But for general aviation aircraft that are primarily made from fiber-reinforced plastics (composites), the methods are not as widely known.
And although aluminum is much tougher strictly from a material standpoint, composites can offer many safety and in-service advantages in addition to reducing weight and improving flight performance. But with those advantages comes the need to understand, design, handle and repair composites appropriately. But are composites safer in a crash?
You asked for it. As a follow-up to the composite airframe tech article in the March 2023 Aviation Consumer, here’s a look at composite construction from a crashworthiness view.
CATEGORIZED CRUNCHES
Damage tolerance in composite general aviation airframes can be divided into three major categories: minor incidents like hangar rash, minor accidents like runway or taxiway excursions and major accidents, such as high-speed loss of control or controlled flight into terrain.
Minor dings are just that, although with a composite structure it could be worse than it looks. For routine impact damage—hits from tools dropping on composites during maintenance or luggage banging against the fuselage while being loaded into the plane—the designers primarily rely on the composite material itself to be robust and tough. This type of so-called damage is mostly cosmetic though it can possibly be more serious.
Toughness in a composite is mostly driven by the resin although fiber can play a role (fiberglass tends to be more forgiving of impact damage than carbon). Baseline epoxies are fairly brittle and do not respond we’ll to impact events. Internal plies in the composite can separate (delaminate) and the damage can be difficult to detect.
Because of this limitation, composite airframe structure is typically designed and analyzed with the assumption that a certain number and size of damage features (such as delaminations) exist.
After impact events and during annual inspections, composite surfaces need to be visually inspected for barely visible impact damage (BVID). If dents, dings, scrapes or other defects are visually apparent, additional testing such as ultrasonics or tap testing may be required to determine the extent of the damage. Repairs at this level may be as simple as a resin fill of the dent or a resin injection to fill the delamination.
By using toughened epoxy resin systems, the impact resistance of the composite is significantly improved and the appearance of BVID greatly reduced. Additionally, smart usage of woven fabrics in proper orientations on outer surfaces as we’ll as sufficient thickness of facesheets (thin composite over foam or honeycomb) and proper fibers may help improve durability and damage resistance.
MINOR ACCIDENTS
Pushing your plane into the hangar without checking to see if that tool cart has been put properly away, or even overshooting a runway on landing at slower speeds, can result in minor to substantial damage to the composite structure, but generally is not normally life-threatening.
For this type of damage event, composite airframes rely on design features in the parts themselves to help mitigate risk. Parts may crumple and break while absorbing enough energy to protect more vital structural members. If you ram a wingtip into a hangar by taxiing too close to it, you may need to replace the wingtip fairing, but the wing skins and primary load-bearing spars will likely be perfectly fine.
Depending on the composite part, repairs may be possible or it may make more economic sense to replace the component. Repairs on monolithic composite (composite parts consisting of just the plies of fiber and resin) are relatively straightforward—the extent of the damaged area is determined and removed by grinding or sanding at a shallow angle through the thickness (called a “scarf” joint); new plies are then replaced in the scarf joint and cured.
Fixing sandwich structure (thin skins or “facesheets” of composite over core honeycomb or foam) is a bit trickier. Finding the extent of the damage is only part of the challenge. The facesheets must be removed, the damaged core removed, new core bonded in and then new facesheets cured on.
MAJOR ACCIDENTS
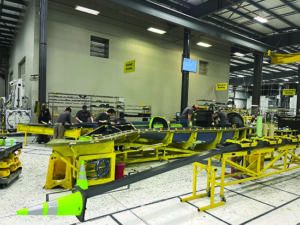
When things get really serious and good landings just aren’t possible, such as with an inflight engine fire, engine failure, loss of control or inadvertent flight into cumulo-granite, you want your aircraft to do two things: keep the structure around yourself and your passengers intact, and prevent or reduce a post-crash fire.
To accomplish the first goal while working with materials (composites) that are significantly less tough than aluminum, airframe designers need to apply careful (and sometimes, very clever) features to absorb energy and provide protection to occupants.
The landing gear struts are designed to absorb everyday landing energy and hard landings and even (in fixed-gear planes) serve as energy absorption features in crash landings or BRS (ballistic recovery systems or whole-airplane parachutes) touchdowns by flexing and bending prior to shearing off. Below the seats are additional energy-absorbing materials (high-density foams and/or honeycomb, much like the crash attenuators on guard rails or attached to the back of road crew vehicles).
These function to absorb crash energy and reduce the G-loads that occupants may experience—to lessen the “sudden stop” that is worse than the fall.
Similar to Formula One’s monocoque “survival cell,” the cabins of composite general aviation aircraft are designed with rollover in mind and have structural features to serve as a roll cage to protect occupants. Stiff and strong (but lightweight) carbon fiber canopy frames help protect heads and upper torsos.
Bonded joints are much more typical on composites than aluminum (which are normally mechanically fastened by rivets or bolts), and provide far superior load transfer in crash situations without the peaking of stress around the fasteners. This helps keep the structure intact during high-impact events, helping to protect the occupants.
Robert Kremnitzer at Diamond Aircraft told us the major advantage when designing in composite materials is that one easily can design for specific purposes. Next to aerodynamic performance and strength, durability, repairability and crash safety are such design purposes.
“Putting the material and its specific properties in the right spot significantly increases strength, durability and passive safety with minimal added structural weight,” he noted. He said that some shells or panels use layup up that are beyond the minimum strength requirements simply because of day-to-day interactions with passengers, crew or maintenance staff—all of which can be tough on structures.
Consider, too, that general aviation aircraft are designed to contain engine fires forward of the firewall and give the pilot a fighting chance to get the plane safely on the ground. The firewall needs to be able to handle 2000 degrees F for 15 minutes, which is why many firewalls are combinations of fire-resistance material like ceramics or heat blankets on structural members like stainless steel or higher temperature capable composites.
POST-CRASH FIRE
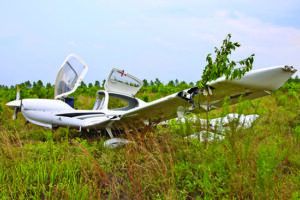
Fires after a crash are mostly due to fuel tank punctures or leakage. Diamond Aircraft has taken an interesting approach to address this specific issue by not using integral fuel tanks. Instead, the design has discrete metal tanks protected between the two wing spars.
This adds weight to the plane, but also gives it additional robustness to eliminate or greatly reduce fuel leakage to minimize the size and duration of any post-crash fire. Perhaps not too surprisingly, based on this focus, no Diamond aircraft showed up in our NTSB/CAROL search for composite airplanes involved in post-crash fires (see sidebar on page 10).
The response to fire in composites can vary widely based on specific resin chemistry and prepreg features. Typically, only materials intended for interior applications (inside the crew/passenger cabin) are tested for FST (fire, smoke and toxicity).
One data point for structural composites is the FAA report cited in our sidebar article. But the resin system that was tested in that report was Boeing’s BMS8-276 used in the 777 and 787; it is not typical of general aviation basic epoxy resins, which are often not self-extinguishing without additives.
However, the basic concept of the report that carbon fiber composites maintain structural integrity longer than aluminum in fire is probably applicable and contributes to the safety of the composite airframe.
Additionally, composites in a fire situation can generate large amounts of smoke and toxic gases (primarily carbon monoxide). Survivors of the crash will want to get out of the cabin as soon as possible and move upwind.
SUMMARY
The way we see it, by using a rigorous and focused systems approach, general aviation designers can use composites to reduce weight and improve performance while burning less fuel, while at the same time designing around the inherent relative brittleness of composites (compared to aluminum) to improve damage tolerance, durability and safety.
Aviation Consumer contributor Gary Bond is a composites Technical Fellow for Solvay—a composite material supplier. He retired from Boeing as a Technical Fellow, and owns and flies a Grumman Tiger.